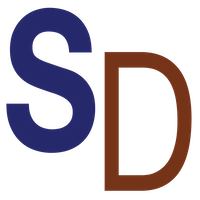
Scientists have taken the clearest picture yet of electronic particles that make up a mysterious magnetic state called quantum spin liquid (QSL).
The achievement could facilitate the development of superfast quantum computers and energy-efficient superconductors.
The scientists are the first to capture an image of how electrons in a QSL decompose into spin-like particles called spinons and charge-like particles called chargons.
“Other studies have seen various footprints of this phenomenon, but we have an actual picture of the state in which the spinon lives. This is something new,” said study leader Mike Crommie, a senior faculty scientist at Lawrence Berkeley National Laboratory (Berkeley Lab) and physics professor at UC.
“Spinons are like ghost particles. They are like the Big Foot of quantum physics — people say that they’ve seen them, but it’s hard to prove that they exist,” said co-author Sung-Kwan Mo, a staff scientist at Berkeley Lab’s Advanced Light Source. “With our method we’ve provided some of the best evidence to date.”
A surprise catch from a quantum wave
In a QSL, spinons freely move about carrying heat and spin — but no electrical charge. To detect them, most researchers have relied on techniques that look for their heat signatures.
Now, as reported in the journal Nature Physics, Crommie, Mo, and their research teams have demonstrated how to characterize spinons in QSLs by directly imaging how they are distributed in a material.
To begin the study, Mo’s group at Berkeley Lab’s Advanced Light Source (ALS) grew single-layer samples of tantalum diselenide (1T-TaSe2) that are only three-atoms thick. This material is part of a class of materials called transition metal dichalcogenides (TMDCs). The researchers in Mo’s team are experts in molecular beam epitaxy, a technique for synthesizing atomically thin TMDC crystals from their constituent elements.
Mo’s team then characterized the thin films through angle-resolved photoemission spectroscopy, a technique that uses X-rays generated at the ALS.
Using a microscopy technique called scanning tunneling microscopy (STM), researchers in the Crommie lab — including co-first authors Wei Ruan, a postdoctoral fellow at the time, and Yi Chen, then a UC Berkeley graduate student — injected electrons from a metal needle into the tantalum diselenide TMDC sample.
Images gathered by scanning tunneling spectroscopy (STS) — an imaging technique that measures how particles arrange themselves at a particular energy — revealed something quite unexpected: a layer of mysterious waves having wavelengths larger than one nanometer (1 billionth of a meter) blanketing the material’s surface.
“The long wavelengths we saw didn’t correspond to any known behavior of the crystal,” Crommie said. “We scratched our heads for a long time. What could cause such long wavelength modulations in the crystal? We ruled out the conventional explanations one by one. Little did we know that this was the signature of spinon ghost particles.”
How spinons take flight while chargons stand still
With help from a theoretical collaborator at MIT, the researchers realized that when an electron is injected into a QSL from the tip of an STM, it breaks apart into two different particles inside the QSL — spinons (also known as ghost particles) and chargons. This is due to the peculiar way in which spin and charge in a QSL collectively interact with each other. The spinon ghost particles end up separately carrying the spin while the chargons separately bear the electrical charge.
In the current study, STM/STS images show that the chargons freeze in place, forming what scientists call a star-of-David charge-density-wave. Meanwhile, the spinons undergo an “out-of-body experience” as they separate from the immobilized chargons and move freely through the material, Crommie said. “This is unusual since in a conventional material, electrons carry both the spin and charge combined into one particle as they move about,” he explained. “They don’t usually break apart in this funny way.”
Crommie added that QSLs might one day form the basis of robust quantum bits (qubits) used for quantum computing. In conventional computing a bit encodes information either as a zero or a one, but a qubit can hold both zero and one at the same time, thus potentially speeding up certain types of calculations. Understanding how spinons and chargons behave in QSLs could help advance research in this area of next-gen computing.
Another motivation for understanding the inner workings of QSLs is that they have been predicted to be a precursor to exotic superconductivity. Crommie plans to test that prediction with Mo’s help at the ALS.
“Part of the beauty of this topic is that all the complex interactions within a QSL somehow combine to form a simple ghost particle that just bounces around inside the crystal,” he said. “Seeing this behavior was pretty surprising, especially since we weren’t even looking for it.”