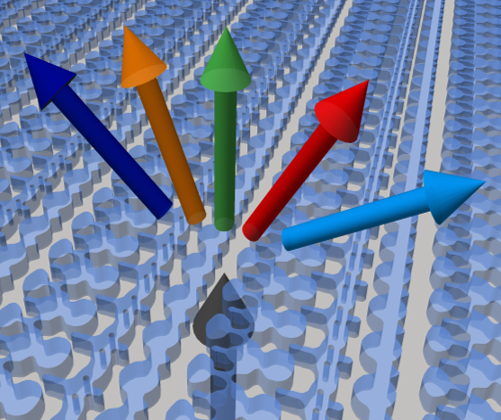

Necessity of antenna miniaturization in wireless communication
In almost all areas of electrical engineering the research interest has been shifted toward miniaturization. Electromagnetism and antennas in particular, are no exception. With the rapid development in wireless systems all over the world, miniaturized antennas have become an active area of research for many antenna engineers. In past several years, a large emphasis has been placed towards miniaturized antenna design. There are many reasons for using small antennas, most of which are physical constraints. For example, high speed aircraft or warships are easy to be damaged due to mechanical strength i.e. wind age for using large antennas. There is a rising interest in making antennas small enough to ultimately fit on a single chip with the rest of the receiving front-end for local area networks. Due to limited space availability for antenna placement in the mobile platforms more antennas can be closely packed that generates the risk of mutual and parasitic coupling between them. Small antennas are required in many applications i.e. invisible military ground vehicles, concealed electrical devices of police or security forces, obstacle clearance on road vehicles or trains, and radio tracking of small animals etc. It is also used in a very recent application like personal communications and handheld devices such as smart phones, tablets, and computers RFID and sensing systems, including printed circuit board design etc.
Effect of miniaturization on Antenna Performances
It is well established that the miniaturizing of an antenna affects its radiation characteristics. Reducing an antenna’s size influence its bandwidth, gain, efficiency, and matching with the feed. This follows directly from the fact that an antenna is a device used to transform a guided wave into a radiated wave, or vice-versa. This transformation process is related to the wavelength, and the antenna size is more significant than the antenna technology in determining how well and for which frequencies this transformation is satisfactory. Many authors have studied the relationship between the electrical dimension of an antenna and its radiation performances for several years. Antenna size and its performance are strongly related to each other. The first fundamental results showing the link between antenna size and its maximum bandwidth and gain were presented in the late 1940s. Wheeler used the radiation power factor, defined as the quotient between the antenna resistance and the antenna reactance, to quantify the radiation of an antenna. Using a simple equivalent lumped circuit, Wheeler deduced that this quotient was equivalent to the bandwidth multiplied by the efficiency, in those cases where the antenna was matched by a simple circuit. This early paper was a first attempt to confirm mathematically the product of efficiency and bandwidth is directly related to the volume occupied by an antenna. Indeed, as the size of an antenna decreases, its reactance increases, but its radiation resistance decreases. The work of Wheeler was generalized by Chu, who derived the equivalent lumped-circuit element from an expansion in partial fractions of the spherical-wave impedance functions around the antenna. Thus, the relationship between the minimum quality factor, Q, of an omnidirectional antenna and its volume was formally established. These results suggest that antenna miniaturization affects gain, bandwidth, and efficiency of the antenna. In addition, due to an increase in antenna Q with a reduction in their electrical size, the antennas become more difficult to match with the feed.
Literature Review
The simplest way to construct a miniaturized antenna is by loading different lumped elements. The idea is that an antenna smaller than a half-wavelength has strong reactive input impedance, which is to be compensated by the loading. The loading can be performed by adding capacitor, coil, top loading etc. The effect of this loading is to either reduce the efficiency of the antenna or to increase the quality factor of the antenna and to thus reduce its bandwidth. A meandered antenna is another simple form of miniaturized antennas where a half wavelength dipole was made compact by meandering the wire. A similar approach had been applied to design a meander type slot antenna. The meandered antennas are very difficult to match with a 50 W line. This difficulty is due to the fact that the radiation of almost in-phase electric currents flowing in opposite directions on closely spaced wires tends to cancel each other in the far-field region. This leads to a very low radiation resistance. In a two-strip meandered line was used to increase the bandwidth of the miniaturized antenna.
The development of metamaterials opened a new possibility for antenna designers to create a novel structure with unconventional properties or enhanced performance. Metamaterials are artificially fabricated structures or media that exhibit unusual properties not found in naturally occurring materials .It can be synthesized by embedding specific inclusions of periodic structures in the host media .Some of these materials exhibit either negative permittivity or negative permeability. If both permittivity and permeability of such materials are negative at the same frequency, then the composite possesses an effective negative index of refraction for isotropic medium and is referred to as a left handed metamaterial. In 1968, first theoretical studies was done by Veselago. After a long gap of his work, there has been a renewed interest in this area with the initiation of the first metamaterial by pendry et al . Pendry proposed an artificial material consisting of the so-called Slit-Ring Resonators(SRR) which exhibit a band of negative permeability values in spite of being made of nonmagnetic materials, and wires which provide the negative permittivity. Next, Smith et al. demonstrated the realization of the first left-handed material which was consisting of an array of SRRs and wires.
Breakthroughs in the field of nanophotonics — how light behaves on the nanometer scale — have paved the way for the invention of “metamaterials,” man-made materials that have enormous applications, from remote nanoscale sensing to energy harvesting and medical diagnostics. But their impact on daily life has been hindered by a complicated manufacturing process with large margins of error.
Now a new interdisciplinary Tel Aviv University study published in Light: Science and Applications demonstrates a way of streamlining the process of designing and characterizing basic nanophotonic, metamaterial elements. The study was led by Dr. Haim Suchowski of TAU’s School of Physics and Astronomy and Prof. Lior Wolf of TAU’s Blavatnik School of Computer Science and conducted by research scientist Dr. Michael Mrejen and TAU graduate students Itzik Malkiel, Achiya Nagler and Uri Arieli.
“The process of designing metamaterials consists of carving nanoscale elements with a precise electromagnetic response,” Dr. Mrejen says. “But because of the complexity of the physics involved, the design, fabrication and characterization processes of these elements require a huge amount of trial and error, dramatically limiting their applications.”
“Our new approach depends almost entirely on Deep Learning, a computer network inspired by the layered and hierarchical architecture of the human brain,” Prof. Wolf explains. “It’s one of the most advanced forms of machine learning, responsible for major advances in technology, including speech recognition, translation and image processing. We thought it would be the right approach for designing nanophotonic, metamaterial elements.”
The scientists fed a Deep Learning network with 15,000 artificial experiments to teach the network the complex relationship between the shapes of the nanoelements and their electromagnetic responses. “We demonstrated that a ‘trained’ Deep Learning network can predict, in a split second, the geometry of a fabricated nanostructure,” Dr. Suchowski says.
The researchers also demonstrated that their approach successfully produces the novel design of nanoelements that can interact with specific chemicals and proteins.
“These results are broadly applicable to so many fields, including spectroscopy and targeted therapy, i.e., the efficient and quick design of nanoparticles capable of targeting malicious proteins,” says Dr. Suchowski. “For the first time, a novel Deep Neural Network, trained with thousands of synthetic experiments, was not only able to determine the dimensions of nanosized objects but was also capable of allowing the rapid design and characterization of metasurface-based optical elements for targeted chemicals and biomolecules.
“Our solution also works the other way around. Once a shape is fabricated, it usually takes expensive equipment and time to determine the precise shape that has actually been fabricated. Our computer-based solution does that in a split second based on a simple transmission measurement.”
The researchers, who have also written a patent on their new method, are currently expanding their Deep Learning algorithms to include the chemical characterization of nanoparticles.
This article has been republished from materials provided by AFTAU. Note: material may have been edited for length and content. For further information, please contact the cited source.
Reference: Malkiel, I., Mrejen, M., Nagler, A., Arieli, U., Wolf, L., & Suchowski, H. (2018). Plasmonic nanostructure design and characterization via Deep Learning. Light: Science & Applications, 7(1), 60. https://doi.org/10.1038/s41377-018-0060-7